Scientists were able to fashion ultrathin silicon nanoantennas that can trap and redirect light at will, similar to how echo chambers work with sound.
A team from Stanford University achieved an approach to significantly slowing light down. Ultrathin silicon chips were used to fabricate resonant nanoscale bars that can trap and redirect light. The results of their study were first published last Monday, August 17, in Nature Nanotechnology.
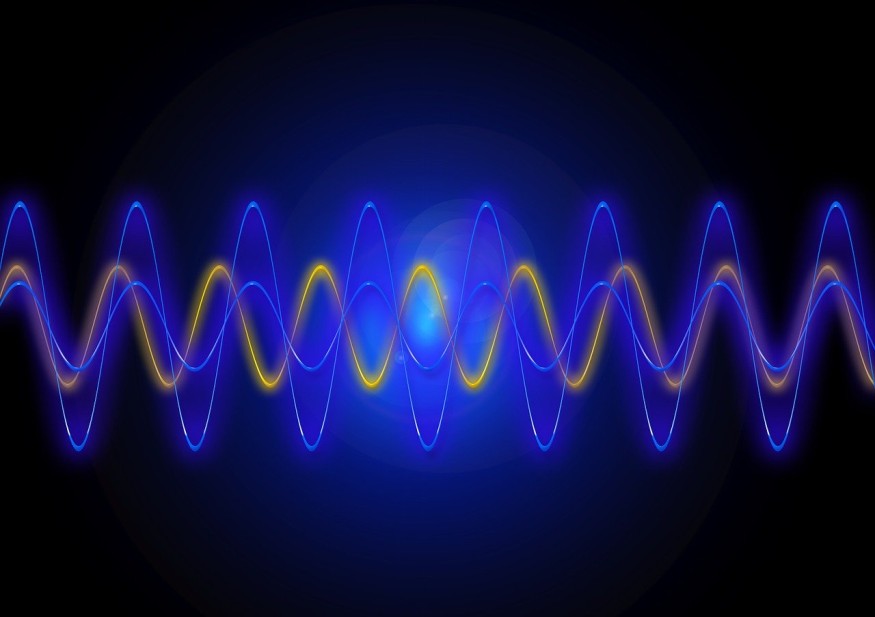
Generating High Q-Factor Resonance
In physics and materials engineering, the quality factor (Q-factor) describes how underdamped a resonator or an oscillator is. It is often used as a parameter to quantify the performance of electronic components, and by extension, circuits.
RELATED: New Photodetector Exceeds 100% Efficiency
Regarding the Stanford University project, they were able to achieve these high Q-factor resonators with the ultrathin silicon nanoantennas—extremely thin strands of silicon. These nanoantennas are installed on top of a transparent wafer base, constructed from sapphire in the experimental setup. Then, researchers etched the nanoantenna pattern using an electron microscope "pen." The silicon nanoantenna is the analog for the soundproof walls in an echo chamber. In this case, however, the slightest imperfection in the etching process.
"High-Q resonances require the creation of extremely smooth sidewalls that don't allow the light to leak out," said Jennifer Dionne, Associate Professor of Materials Science and Engineering at Stanford and the Senior Associate Vice Provost of Research Platforms/Shared Facilities. She added that these structures are reasonably achievable with larger structures on the micron scale. However, with nanostructures, which are smaller and scatter more light, achieving the same results can be "challenging."
"We're essentially trying to trap light in a tiny box that still allows the light to come and go from many different directions," added Mark Lawrence, postdoctoral fellow and lead author of the study. "It's easy to trap light in a box with many sides, but not so easy if the sides are transparent—as is the case with many Silicon-based applications," Lawrence explained.
High Q-Factor Applications
In their experiments, the Stanford University team was able to attain Q-factors of up to 2,500. This is two orders of magnitude, or 100 times, higher than what is possible with existing technology. In light applications, the quality factor is used to quantify resonance behavior, which is proportional to the lifetime of the light. Dionne said that by achieving q-factors in the thousands order, they are already in "a nice sweet spot from some very exciting technological applications."
Finding a novel approach to the control and manipulation of light has potential game-changing applications—from quantum computing, virtual reality (VR) programming, light detection and ranging (LiDAR), and communications. It can even be applied in detecting viruses, like SARS-CoV-2.
RELATED: New Quantum Key Distribution Protocol Maintains Security Over Long Distances
One example noted in the Stanford University news article is in biosensing, posing the difficulty of detecting the virtually invisible biomolecules. A controlled light that can penetrate the molecule by up to thousands of times could make the target more detectable, as the target will most likely scatter light upon contact.
Dionne's laboratory is working on using this technology in detecting COVID-19 antigens and antibodies, relying on the strong light-molecule interaction to allow the detection of these small materials that aid the immune response against the disease. They added that it is possible to independently control each of the antennas to search for a particular antibody.
Stanford researchers have fashioned ultrathin silicon nanoantennas that trap and redirect light, for potential applications in quantum computing, virtual reality and augmented reality; light-based WiFi; and even the detection of viruses like SARS-CoV-2. https://t.co/6HofZ3l7ha — Stanford University (@Stanford) August 19, 2020
Check out more news and information on Materials Engineering on Science Times.