The threat of climate change and its irrevocable negative implications on our ecosystems have pushed researchers to scour the globe for alternative sources of energy that will allow humans to continue forward without damaging the planet we live on.
By utilizing energy derived from wind turbines and solar modules, water's chemistry can be split via electrolysis into hydrogen and oxygen without producing dangerous byproducts and emissions. Since the availability of renewable energy varies when producing green and neutral hydrogen, it is imperative to understand the behavior of the catalysts under high and dynamic conditions.
What is Green Hydrogen?
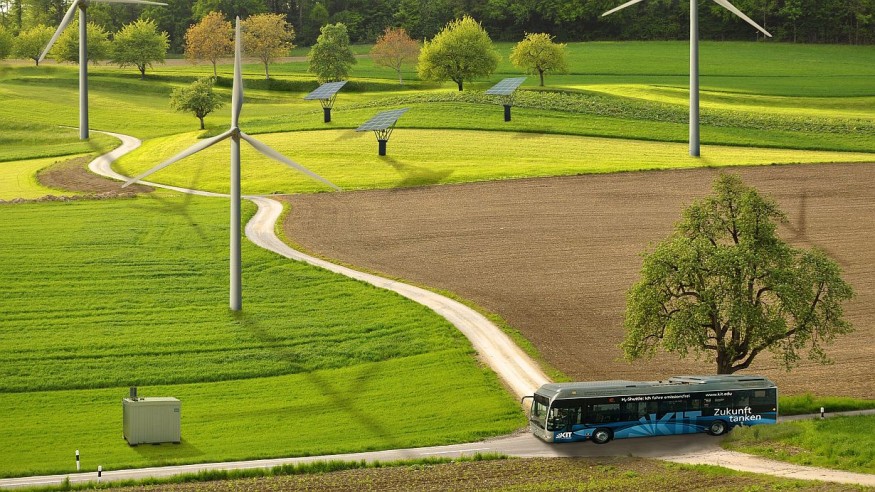
The most abundant element in the universe is Hydrogen; however, here on Earth, it doesn't occur purely in nature and requires energy to be separated and purified. Commonly, hydrogen is extracted from water which has two parts of hydrogen, hence H2O. This is a fairly simple procedure where one can use chemistry and heat principles to release hydrogen from various organic materials like fossil fuels.
On the other hand, this is enormously polluting. Production of hydrogen worldwide is responsible for about the equivalent CO2 emissions of Indonesia, and the United Kingdom combined. Commonly, hydrogen is used in producing ammonia fertilizers and in the oil refining industry.
Luckily, green hydrogen is a cleaner way of extracting pure substances without using fossil fuels. Bypassing a strong electrical current through a water tank splits the chemical makeup of the molecule known as electrolysis. If the electricity used is generated via renewable energy sources producing hydrogen will render no greenhouse gas emission, according to ABC News.
RELATED ARTICLE : Copper Atoms Kept Closer to Combat Bacteria, New Approach Shown in Study
Catalysis, The Hope for Green Hydrogen
KIT's Institute for Chemical Technology and Polymer Chemistry expert and lead of the study Steffen Czioska said that anodes could be utilized to observe and precisely measure the intensity of oxygen bubbles on high currents. The experts can conduct a comprehensive investigation of the iridium oxide catalyst, its features, and how it works in dynamic environments. the whole coverage of the study was presented in the ACS Publications, titled "Increased Ir-Ir Interaction in Iridium Oxide during the Oxygen Evolution Reaction at High Potentials Probed by Operando Spectroscopy."
The catalyst. based on the study, was first observed regardless of the strong bubble evolution. With that said, the advancement of the research was considered an essential piece by The American Chemical Society or ACS and even recommended the paper of KIT's experts as a prestigious ACS Editor's Choice.
The catalysis was investigated through the collaborative efforts from the Electrochemical Technologies Group of the Institute for Applied Materials and the Institute of Catalysis Research and Technology. The community groups used a multiple analysis approach with X-ray spectroscopy in the hope of bringing the most accurate results from the experiment.
The study revealed that high voltages and dynamic loading result in an unusual modification in the catalyst's structure and its stabilization. Czisoaka mentioned in a PhysOrg report that lesser iridium oxide causes the specific material to stabilize itself. Due to the results gathered from the catalyst surface and its structure, further studies will be conducted soon to provide better and efficient catalysis in the future of renewable energy production.
RELATED ARTICLE : Ion Dynamics in Phosphate Glass During Hydration; Silicophosphate Potential Solution to Bone and Muscle Disorders
Check out more news and information on Chemistry in Science Times.